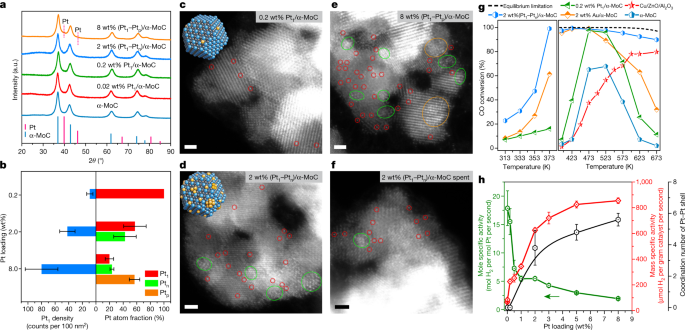
Zhai, Y. et al. Alkali-stabilized Pt-OHx species catalyze low-temperature water–gas shift reactions. Science 329, 1633–1636 (2010).
Rodriguez, J. A. et al. Activity of CeOx and TiOx nanoparticles grown on Au(111) in the water–gas shift reaction. Science 318, 1757–1760 (2007).
Ladebeck, J. R. & Wagner, J. P. in Handbook of Fuel Cells (eds Vielstich, W., Lamm, A. & Gasteiger, H. A.) Ch. 16, 197 (Wiley, 2003).
Yao, S. et al. Atomic-layered Au clusters on α-MoC as catalysts for the low-temperature water–gas shift reaction. Science 357, 389–393 (2017).
Yang, M. et al. A common single-site Pt(ii)–O(OH)x– species stabilized by sodium on “active” and “inert” supports catalyzes the water–gas shift reaction. J. Am. Chem. Soc. 137, 3470–3473 (2015).
Fu, Q., Saltsburg, H. & Flytzani-Stephanopoulos, M. Active nonmetallic Au and Pt species on ceria-based water–gas shift catalysts. Science 301, 935–938 (2003).
Yang, M. et al. Catalytically active Au–O(OH)x– species stabilized by alkali ions on zeolites and mesoporous oxides. Science 346, 1498–1501 (2014).
Zugic, B. et al. Probing the low-temperature water–gas shift activity of alkali-promoted platinum catalysts stabilized on carbon supports. J. Am. Chem. Soc. 136, 3238–3245 (2014).
Ding, K. et al. Identification of active sites in CO oxidation and water–gas shift over supported Pt catalysts. Science 350, 189–192 (2015).
Schweitzer, N. M. et al. High activity carbide supported catalysts for water gas shift. J. Am. Chem. Soc. 133, 2378–2381 (2011).
Li, Z. et al. Reactive metal–support interactions at moderate temperature in two-dimensional niobium-carbide-supported platinum catalysts. Nat. Catal. 1, 349–355 (2018).
Hunt, S. T. et al. Self-assembly of noble metal monolayers on transition metal carbide nanoparticle catalysts. Science 352, 974–978 (2016).
Lu, J., Aydin, C., Browning, N. D. & Gates, B. C. Hydrogen activation and metal hydride formation trigger cluster formation from supported iridium complexes. J. Am. Chem. Soc. 134, 5022–5025 (2012).
Fierro-Gonzalez, J. C. & Gates, B. C. Mononuclear AuIII and AuI complexes bonded to zeolite NaY: catalysts for CO oxidation at 298 K. J. Phys. Chem. B 108, 16999–17002 (2004).
Lu, J., Aydin, C., Browning, N. D. & Gates, B. C. Imaging isolated gold atom catalytic sites in zeolite NaY. Angew. Chem. Int. Ed. 51, 5842–5846 (2012).
Miller, J. T. et al. The effect of gold particle size on Au–Au bond length and reactivity toward oxygen in supported catalysts. J. Catal. 240, 222–234 (2006).
Dong, J. et al. Carbide-supported Au catalysts for water–gas shift reactions: a new territory for the strong metal–support interaction effect. J. Am. Chem. Soc. 140, 13808–13816 (2018).
Sabnis, K. D. et al. Water–gas shift catalysis over transition metals supported on molybdenum carbide. J. Catal. 331, 162–171 (2015).
Lin, L. et al. Low-temperature hydrogen production from water and methanol using Pt/α-MoC catalysts. Nature 544, 80–83 (2017).
Lin, J. et al. Remarkable performance of Ir1/FeOx single-atom catalyst in water gas shift reaction. J. Am. Chem. Soc. 135, 15314–15317 (2013).
Fu, Q., Deng, W., Saltsburg, H. & Flytzani-Stephanopoulos, M. Activity and stability of low-content gold-cerium oxide catalysts for the water–gas shift reaction. Appl. Catal. B 56, 57–68 (2005).
Murugappan, K. et al. Operando NAP-XPS unveils differences in MoO3 and Mo2C during hydrodeoxygenation. Nat. Catal. 1, 960–967 (2018).
Porosoff, M. D., Yang, X., Boscoboinik, J. A. & Chen, J. G. Molybdenum carbide as alternative catalysts to precious metals for highly selective reduction of CO2 to CO. Angew. Chem. Int. Ed. 53, 6705–6709 (2014).
Prosvirin, I. P., Bukhtiyarov, A. V., Bluhm, H. & Bukhtiyarov, V. I. Application of near ambient pressure gas-phase X-ray photoelectron spectroscopy to the investigation of catalytic properties of copper in methanol oxidation. Appl. Surf. Sci. 363, 303–309 (2016).
Mudiyanselage, K. et al. Importance of the metal-oxide interface in catalysis: in situ studies of the water–gas shift reaction by ambient-pressure X-ray photoelectron spectroscopy. Angew. Chem. Int. Ed. 52, 5101–5105 (2013).
Lin, L. et al. A highly CO-tolerant atomically dispersed Pt catalyst for chemoselective hydrogenation. Nat. Nanotechnol. 14, 354–361 (2019).
Namiki, T., Yamashita, S., Tominaga, H. & Nagai, M. Dissociation of CO and H2O during water–gas shift reaction on carburized Mo/Al2O3 catalyst. Appl. Catal. A 398, 155–160 (2011).
Kalamaras, C. M., Americanou, S. & Efstathiou, A. M. “Redox” vs “associative formate with –OH group regeneration” WGS reaction mechanism on Pt/CeO2: effect of platinum particle size. J. Catal. 279, 287–300 (2011).
Deng, W., Carpenter, C., Yi, N. & Flytzani-Stephanopoulos, M. Comparison of the activity of Au/CeO2 and Au/Fe2O3 catalysts for the CO oxidation and the water–gas shift reactions. Top. Catal. 44, 199–208 (2007).
Yang, M., Allard, L. F. & Flytzani-Stephanopoulos, M. Atomically dispersed Au– (OH)x species bound on titania catalyze the low-temperature water–gas shift reaction. J. Am. Chem. Soc. 135, 3768–3771 (2013).
de la Peña, F. et al. hyperspy/hyperspy: HyperSpy v1.5.2. https://doi.org/10.5281/zenodo.3396791 (2019).
Herzing, A. A. et al. Identification of active gold nanoclusters on iron oxide supports for CO oxidation. Science 321, 1331–1335 (2008).
Artiglia, L. et al. Introducing time resolution to detect Ce3+ catalytically active sites at the Pt/CeO2 interface through ambient pressure X-ray photoelectron spectroscopy. J. Phys. Chem. Lett. 8, 102–108 (2017).
Orlando, F. et al. The environmental photochemistry of oxide surfaces and the nature of frozen salt solutions: a new in situ XPS approach. Top. Catal. 59, 591–604 (2016).
"low" - Google News
January 20, 2021 at 11:27PM
https://ift.tt/39QRFsw
A stable low-temperature H2-production catalyst by crowding Pt on α-MoC - Nature.com
"low" - Google News
https://ift.tt/2z1WHDx
Bagikan Berita Ini
0 Response to "A stable low-temperature H2-production catalyst by crowding Pt on α-MoC - Nature.com"
Post a Comment